By Lee
Smolin | reprinted from the Jan/Feb 2006 issue.
For more than two hundred years, we physicists have been on a
wild ride. Our search for the most fundamental laws of nature has
been rewarded by a continual stream of discoveries. Each decade back
to 1800 saw one or more major additions to our knowledge about
motion, the nature of matter, light and heat, space and time. In the
20th century, the pace accelerated dramatically.
Then, about 30 years ago, something changed. The last time there
was a definitive advance in our knowledge of fundamental physics was
the construction ofthe theory we call the standard model of
particle physics in 1973. The last time a fundamental theory was
proposed that has since gotten any support from experiment was a
theory about the very early universe called inflation, which
was proposed in 1981.
Since then, many ambitious theories have been invented and
studied. Some of them have been ruled out by experiment. The rest
have, so far, simply made no contact with experiment. During the
same period, almost every experiment agreed with the predictions of
the standard model. Those few that didn't produced results so
surprising—so unwanted—that baffled theorists are still unable to
explain them.
The growing gap between theory and experiment is not due to a
lack of big open problems. Much of our work since the 1970s has been
driven by two big questions: 1) Can we combine quantum theory and
general relativity to make a quantum theory of gravity? and 2) Can
we unify all the particles and forces, and so understand them in
terms of a simple and completely general law? Other mysteries have
deepened, such as the question of the nature of the mysterious dark
energy and dark matter.
Traditionally, physics progressed by acontinual interplay of
theory and experiment. Theorists hypothesized ideas and principles,
which were explored by stating them in precise mathematical
language. This allowed predictions to be made, which
experimentalists then test. Conversely, when there is a surprising
new experimental finding, theorists attempt to model it in order to
test the adequacy of the current theories.
There appears to be no precedent for a gap between theory and
experiment lasting decades (see timeline). It is something we
theorists talk about often. Some see it as a temporary lull and look
forward to new experiments now in preparation. Others speak of a new
era in science in which mathematical consistency has replaced
experiment as the final arbiter of a theory's correctness. A growing
number of theoretical physicists, myself among them, see the present
situation as a crisis that requires us to reexamine the assumptions
behind our so-far unsuccessful theories.
I should emphasize that this crisis involves only fundamental
physics—that part of physics concerned with discovering the laws
of nature. Most physicist sare concerned not with this but with
applying the laws we know to understandand control myriads of
phenomena. Those are equally important endeavors, and progress in
these domains is healthy.
Contending theories
Since the 1970s, many theories of unification have been
proposedand studied, going under fanciful names such as preonmodels,
technicolor, supersymmetry, braneworlds, and, most popularly, string
theory. Theories of quantum gravity include twistor theory, causal
set models, dynamical triangulation models, and loop quantum
gravity. One reason string theory is popular is that there is some
evidence that it points to a quantum theory of gravity.
Has physics reached an impasse, and what can
we do about it?
One source of the crisis is that many of these theories have many
freely adjustable parameters. As a result, some theories make no
predictions at all. But even in the cases where they make a
prediction, it is not firm. If the predicted new particle or effect
is not seen, theorists can keep the theory alive by changing the
value of a parameter to make it harder to see in experiment.
The standard model of particle physics has about 20 freely
adjustable parameters, whose values were set by experiment.
Theorists have hoped that a deeper theory would provide explanations
for the values the parameters are observed to take. There has been a
naive, but almost universal, belief that the more different forces
and particles are unified into a theory, the fewer freely adjustable
parameters the theory will have.
This is not the way things have turned out. There are theories
that have fewer parameters than the standard model, such as
technicolor and preon models. But it has not been easy to get them
to agree with experiment. The most popular theories, such as
supersymmetry, have many more free parameters—the simplest
supersymmetric extension of the standard model has 105 additional
free parameters. This means that the theory is unlikely to be
definitively tested in upcoming experiments. Even if the theory is
not true, many possible outcomes of the experiments could be made
consistent with some choice of the parameters of the theory.
String theory comes in a countably infinite number of versions,
most of which have many free parameters. String theorists speak no
longer of a single theory, but of a vast "landscape1"
of possible theories. Moreover, some cosmologists argue for an
infinity of universes, each of which is governed by a different
theory. A tiny fraction of these theories may be roughly compatible
with present observation, but this is still a vast number, estimated
to be greater than 10400 theories. (Nevertheless, so far
not a single version consistent with all experiments has been
written down.) No matter what future experiments see, the results
will be compatible with vast numbers of theories, making it unlikely
that any experiment could either confirm or falsify string
theory.
This realization has brought the present crisis to a head. Steven
Weinberg and Leonard Susskind have argued for a new definition of
science in which a theory maybe believed without being subject to a
definitive experiment whose result could kill it. Some theorists
even tell us we are faced with a choice of giving up string
theory—which is widely believed by theorists—or giving up our
insistence that scientific theories must be testable. As Steven
Weinberg writes in a recent essay2:
Most advances in the history of science have been
marked by discoveries about nature, but at certain turning points
we have made discoveries about science itself ... Now we may
be at a new turning point, a radical change in what we accept as a
legitimate foundation for a physical theory ... The larger
the number of possible values of physical parameters provided by
the string landscape, the more string theory legitimates anthropic
reasoning as a new basis for physical theories: Any scientists who
study nature must live in a part of the landscape where physical
parameters take values suitable for the appearance of life and its
evolution into scientists.
Among an infinity of theories and an infinity of universes, the
only predictions we can make stem from the obvious fact that we must
live in a universe hospitable to life. If this is true, we will not
be able to subject our theories to experiments that might either
falsify or count as confirmation of them. But, say some proponents
of this view, if this is the way the world is, it's just too bad for
outmoded ways of doing science. Such a radical proposal by such
justly honored scientists requires a considered response.
I believe we should not modify the basic methodological
principles of science to save a particular theory—even a theory that
the majority of several generations of very talented theorists have
devoted their careers to studying. Science works because it is based
on methods that allow well-trained people of good faith, who
initially disagree, to come to consensus about what can be
rationally deduced from publicly available evidence. One of the most
fundamental principles of science has been that we only consider as
possibly true those theories that are vulnerable to being shown
false by doable experiments.
Contending styles of
research
I think the problem is not string theory, per se. It goes deeper,
to a whole methodology and style of research. The great physicists
of the beginning of the 20th century—Einstein, Bohr, Mach,
Boltzmann, Poincare, Schrodinger, Heisenberg—thought of theoretical
physics as a philosophical endeavor. They were motivated by
philosophical problems, and they often discussed their scientific
problems in the light of a philosophical tradition in which they
were at home. For them, calculations were secondary to a deepening
of their conceptual understanding of nature.
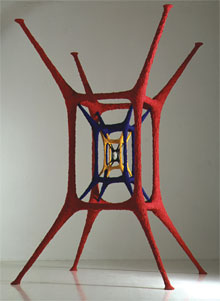 Saint Clair Gemin,
Guardian Angel
|
After the
success of quantum mechanics in the 1920s, this philosophical way of
doing theoretical physics gradually lost out to a more pragmatic,
hard-nosed style of research. This is not because all the
philosophical problems were solved: to the contrary, quantum theory
introduced new philosophical issues, and the resulting controversy
has yet to be settled. But the fact that no amount of philosophical
argument settled the debate about quantum theory went some way to
discrediting the philosophical thinkers. It was felt that while a
philosophical approach may have been necessary to invent quantum
theory and relativity, thereafter the need was for physicists who
could work pragmatically, ignore the foundational problems, accept
quantum mechanics as given, and go on to use it. Those who either
had no misgivings about quantum theory or were able to put their
misgivings to one side were able in the next decades to make many
advances all over physics, chemistry, and astronomy.
The shift to a more pragmatic approach to physics was completed
when the center of gravity of physics moved to the United States in
the 1940s. Feynman, Dyson, Gell-Mann, and Oppenheimer were aware of
the unsolved foundational problems, but they taught a style of
research in which reflection on them had no place in research.
By the time I studied physics in the 1970s, the transition was
complete. When we students raised questions about foundational
issues, we were told that no one understood them, but it was not
productive to think about that. "Shut up and calculate," was the
mantra. As a graduate student, I was told by my teachers that it was
impossible to make a career working on problems in the foundations
of physics. My mentors pointed out that there were no interesting
new experiments in that area, whereas particle physics was driven by
a continuous stream of new experimental discoveries. The one
foundational issue that was barely tolerated, although discouraged,
was quantum gravity.
"I believe we should not modify the basic
methodological principles ofscience to save a particular
theory."
This rejection of careful foundational thought extended to a
disdain for mathematical rigor. Our uses of theories were based on
rough-and-ready calculation tools and intuitive arguments. There was
in fact good reason to believe that the standard model of particle
physics is not mathematically consistent at a rigorous level. As a
graduate student at Harvard, I was taught not to worry about this
because the contact with experiment was more important. The fact
that the predictions were confirmed meant that something was right,
even if there might be holes in the mathematical and conceptual
foundations, which someone would have to fix later.
In retrospect, it seems likely that this style of research, in
which conceptual puzzles and issues of mathematical rigor were
ignored, can only succeed if it is tightly coupled to experiment.
When the contact with experiment disappeared in the 1980s, we were
left with an unprecedented situation. The string theories are
understood, from a mathematical point of view, as badly as the older
theories, and most of our reasoning about them is based on
conjectures that remain unproven after many years, at any level of
rigor. We do not even have a precise definition of the theory,
either in terms of physical principles or mathematics. Nor do we
have any reasonable hope to bring the theory into contact with
experiment in the foreseeable future. We must ask how likely it is
that this style of research can succeed at its goal of discovering
new laws of nature.
It is difficult to find yourself in disagreement with the
majority of your scientific community, let alone with several heroes
and role models. But after a lot of thought I've come to the
conclusion that the pragmatic style of research is failing. By 1980,
we had probably gone as far as we could by following this pragmatic,
antifoundational methodology. If we have failed to solve the key
problems of quantum gravity and unification in a way that connects
to experiment, perhaps these problems cannot be solved using the
style of research tha twe theoretical physicists have become
accustomed to. Perhaps the problems of unification and quantum
gravity are entangled with the foundational problems of quantum
theory, as Roger Penrose and Gerard t'Hooft think. If they are
right, thousands of theorists who ignore the foundational problems
have been wasting their time.
There are approaches to unification and quantum gravity that are
more foundational. Several of them are characterized by a property
we call background independence. This means that the geometry of
space is contingent and dynamical; it provides no fixed background
against which the laws of nature can be defined. General relativity
is background-independent, but standard formulations of quantum
theory—especially as applied to elementary particle physics—cannot
be defined without the specification of a fixed background. For this
reason, elementary particle physics has difficulty incorporating
general relativity.
String theory grew out of elementary particle physics and, at
least so far, has only been successfully defined on fixed
backgrounds. Thus, the infinity of string theories which are known
are each associated with a single space-time background.
Those theorists who feel that theories should be
background-independent tend to be more philosophical, more in
thetradition of Einstein. The pursuit of background-independent
approaches to quantum gravity has been pursued by such
philosophically sophisticated scientists as John Baez, Chris Isham,
Fotini Markopoulou, Carlo Rovelli, and Raphael Sorkin, who are
sometimes even invited to speak at philosophy conferences. This is
not surprising, because the debate between those who think space has
a fixed structure and those who think of it as a network of
dynamical relationships goes back to the disputes between Newton and
his contemporary, the philosopher Leibniz.
Meanwhile, many of those who continue to reject Einstein's legacy
and work with background-dependent theories are particle physicists
who are carrying on the pragmatic, "shut-up-and calculate" legacy in
which they were trained. If they hesitate to embrace the lesson of
general relativity that space and time are dynamical, it may be
because this is a shift that requires some amount of critical
reflection in a more philosophical mode.
Thus, I suspect that the crisis is a result of having ignored
foundational issues. If this is true, the problems of quantum
gravity and unification can only be solved by returning to the older
style of research.
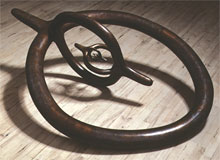 Saint Clair Gemin, Search
for Water
|
How well could
this be expected to turn out? For the last 20 years or so, there has
been a small resurgence of the foundational style of research. It
has taken place mainly outside the United States, but it is
beginning to flourish in a fewcenters in Europe, Canada, and
elsewhere. This style has led to very impressive advances, such as
the invention of the idea of the quantum computer. While this was
suggested earlier by Feynman, the key step that catalyzed the field
was made by David Deutsch, a very independent, foundational thinker
living in Oxford. For the last few years, experimental work on the
foundations of quantum theory has been moving faster than
experimental particle physics. And some leading experimentalists in
this area, such as Anton Zeilinger, in Vienna, talk and write about
their experimental programs in the context of the philosophical
problems that motivate them.
Currently, there is a lot of optimism and excitement among the
quantum gravity community about approaches that embrace the
principle of background independence. One reason is that we have
realized that some current experiments do test aspects of quantum
gravity; some theories are already ruled out and others are to be
tested by results expected soon.
A notable feature of the background independent approaches to
quantum gravity is that they suggest that particle physics, and even
space-time itself, emerge as collective phenomena. This implies a
reversal of the hierarchical way of looking at science, in which
particle physics is the most "fundamental" and mechanisms by which
complex and collective behavior emerge are less fundamental.
So, while the new foundational approaches are still pursued by a
minority of theorists, the promise is quite substantial. We have in
front of us two competing styles of research. One, which 30 years
ago was the way to succeed, now finds itself in a crisis because it
makes no experimental predictions, while another is developing
healthily, and is producing experimentally testable hypotheses. If
history and common sense are any guide, we should expect that
science will progress faster if we invest more in research that
keeps contact with experiment than in a style of research that seeks
to amend the methodology of science to excuse the fact that it
cannot make testable predictions about nature.
1 Smolin, L. 1997. The Life of the
Cosmos. Oxford University Press.
Amazon
| Barnes
& Noble
2 Weinberg,
S. 2005. Living
in the multiverse. arXiv:hep-th/0511037. Preprint.
(PDF, 124 KB) FULL TEXT
Lee Smolin is a theoretical
physicist who has made important contributions to the search for
quantum theory of gravity. He is a founding researcher at the
Perimeter Institute for Theoretical Physics in Waterloo, Ontario. He
is the author of Life of the Cosmos (Oxford, 1997), Three
Roads to Quantum Gravity (Orion, 2001), and the forthcoming,
The Trouble with Physics (Houghton Mifflin, 2006).
The sculptures, The Four, Guardian Angel, and
Search for Water, are reproduced with permission of the
artist, Saint Clair Cemin.